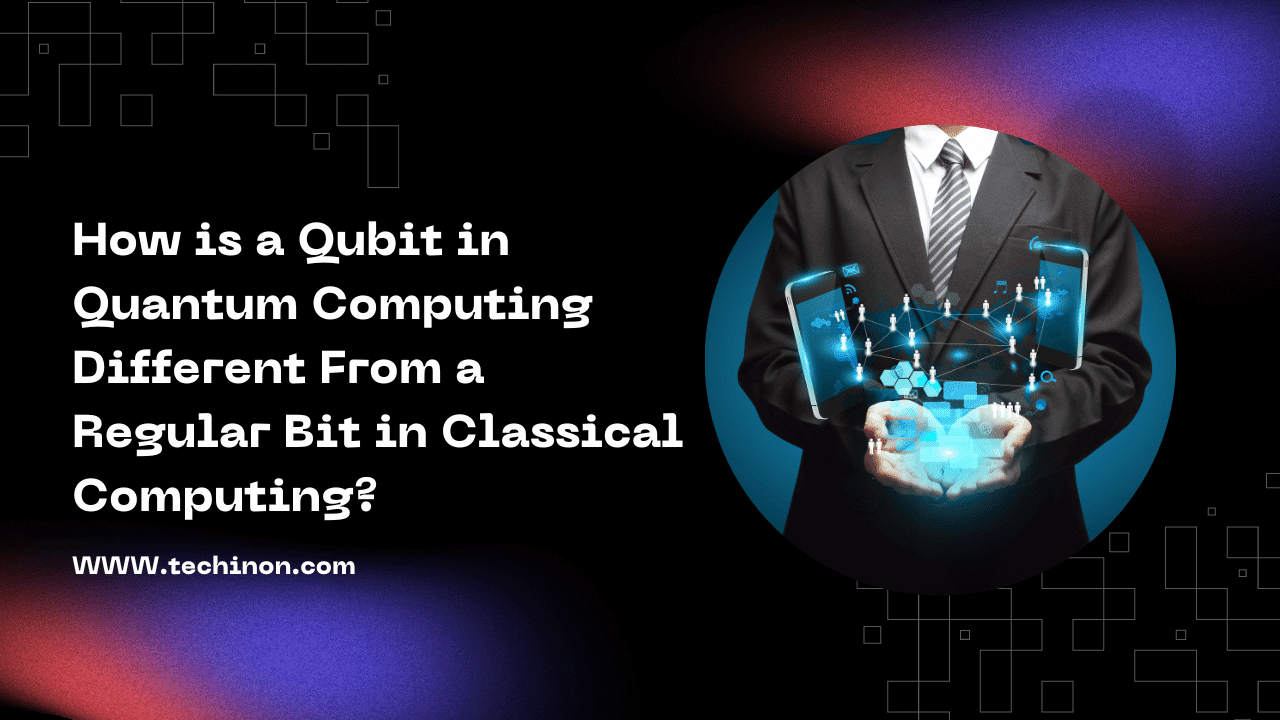
Introduction
In the ever-evolving landscape of technology, one concept has captured the imagination of scientists, engineers, and futurists alike: quantum computing. At the heart of this groundbreaking paradigm shift lies the qubit, a fundamental unit of quantum information. To truly appreciate the marvel of qubits, we must explore their distinctions from classical bits, the cornerstone of classical computing. This article takes you on a journey how is a qubit in quantum computing different from a regular bit in classical computing?
Quantum vs. Classical Computing: A Glimpse into the Future
Classical bits, the building blocks of classical computing, are like the bricks in a well-constructed wall. They represent binary states—0 or 1—forming the basis of logical operations. Quantum computing, however, delves into a realm where these binary shackles are shattered, and qubits emerge as the architects of unparalleled computational prowess.
Qubits and Classical Bits: Unveiling the Essence
At its core, a classical bit can only exist in one of two states: 0 or 1. It’s akin to a light switch, which can be either off or on. Qubits, on the other hand, embrace the magic of superposition, allowing them to inhabit multiple states simultaneously. This remarkable trait enables qubits to perform a multitude of calculations concurrently, propelling quantum computing into a realm of unmatched efficiency.
Quantum Superposition: A Symphony of Possibilities
Imagine standing at a crossroads where you can take every path simultaneously. This is the essence of quantum superposition. While classical bits are confined to a single state at any given time, qubits gracefully pirouette through a mesmerizing dance of possibilities. As a result, they can process a wealth of information concurrently, opening doors to complex problem-solving that classical computers could only dream of achieving.
Entanglement: Quantum Computing’s Love Affair
In the entangled embrace of qubits, quantum computing achieves a marvel that challenges our very understanding of reality. Quantum entanglement, an intricately woven connection between qubits, enables them to communicate instantaneously, regardless of distance. This phenomenon defies the constraints of space and time, granting quantum computers the potential to revolutionize communication and cryptography.
Quantum Parallelism vs. Classical Sequentiality: A Paradigm Shift
In the classical realm, computation marches in a linear procession—a sequential ballet where each step follows the previous one. Quantum parallelism, however, introduces a symphony of simultaneous computations. Qubits, orchestrated in delicate harmony, perform calculations that would overwhelm classical computers with their intricacy. This leap in processing power has profound implications for fields like cryptography, optimization, and artificial intelligence.
Quantum Gates: Orchestrating the Quantum Symphony
In the world of classical computing, logic gates manipulate classical bits to perform computations. Quantum gates, akin to their classical counterparts, choreograph the dance of qubits. Yet, these gates wield a magic that transcends classical limitations. By applying quantum gates, we manipulate qubits, harnessing their superposition and entanglement to solve problems that once seemed insurmountable.
Quantum Measurement: Peering into the Quantum Abyss
As qubits spiral through superposition and entanglement, the moment of measurement beckons—a transformation that collapses their multitude of states into a single outcome. This act of measurement, however, is no ordinary observation. It’s a complex interplay of probabilities, where the quantum world unveils its secrets, yielding solutions that would evade classical computers.
The Delicate Dance of Quantum Coherence
While qubits’ superposition and entanglement propel quantum computing’s power, maintaining coherence within qubits is a challenge. Quantum coherence, a state of delicate harmony, is easily disrupted by external influences—a phenomenon known as decoherence. Researchers tirelessly work on strategies to prolong coherence, safeguarding the fragile quantum states and preserving their computational might.
Quantum Error Correction: Guardians of Quantum Precision
Errors are inevitable in the realm of computing, be it classical or quantum. Yet, quantum error correction unveils an ingenious defense mechanism. By encoding information redundantly across multiple qubits, researchers devise methods to detect and rectify errors, ensuring the fidelity of quantum computations—a feat that reinforces the reliability of quantum algorithms.
Quantum Supremacy: Pioneering a New Era
Quantum supremacy, a concept that dances at the intersection of theory and reality, emerges when a quantum computer completes a task beyond the reach of classical computers. It’s a milestone that showcases the prowess of qubits, heralding an era where complex problems yield to the quantum dance of superposition and entanglement.
Applications: From Enigma to Advancements
The applications of qubits extend far beyond calculations and algorithms. Quantum cryptography leverages qubits’ unique properties to create unbreakable codes, safeguarding communications in a world vulnerable to classical eavesdropping. Quantum simulation transforms complex scientific inquiries into tangible insights, revolutionizing fields from material science to drug discovery.
Quantum Cryptography: Unbreakable Codes and Secure Communications
The art of encryption has always been a critical aspect of communication. Classical cryptography employs mathematical algorithms to encode messages, but it can be vulnerable to advanced computing techniques. This is where qubits step in, ushering in the era of quantum cryptography.
Qubits’ ability to exist in multiple states simultaneously and their entanglement phenomena lay the foundation for unbreakable codes. Quantum key distribution (QKD), a cornerstone of quantum cryptography, enables two parties to establish a secret key for secure communication without the fear of interception or eavesdropping. The very act of eavesdropping on qubits disrupts their delicate quantum states, alerting the communicating parties of any unauthorized interference.
Quantum Simulation: Unraveling Complexity with Qubits
The complexities of our universe extend far beyond our immediate perception. Simulating the behavior of intricate systems, such as molecular interactions or quantum phenomena, often requires immense computational resources. This is where qubits wield their power as computational wizards.
Quantum simulation leverages qubits’ inherent ability to mimic the behavior of complex systems with astonishing accuracy. By encoding the characteristics of the system into qubits and applying quantum gates, scientists can unlock insights that would otherwise remain hidden in the labyrinth of computation. This revolutionizes fields like materials science, enabling the discovery of new materials with tailored properties and revolutionizing drug discovery by simulating molecular interactions at unprecedented levels of detail.
The Road Ahead: Quantum Coherence and Challenges
While the realm of quantum computing is brimming with potential, it’s not devoid of challenges. One of the most significant hurdles is maintaining quantum coherence—the delicate state where qubits exist in superposition and entanglement. External factors, such as temperature fluctuations and electromagnetic interference, can disrupt this coherence, leading to errors and degraded performance.
Researchers tirelessly work to develop techniques that mitigate decoherence, aiming to prolong the lifespan of qubits’ delicate quantum states. Quantum error correction, as mentioned earlier, plays a pivotal role in rectifying errors caused by decoherence. As advancements in materials science and engineering continue, the dream of building fault-tolerant quantum computers—machines capable of performing complex computations reliably—draws closer to reality.
Also Read: Which Situation is a Current Example of a Use Case in Quantum Computing?
Closing Thoughts: Embracing the Quantum Frontier
As we conclude our journey into the enigmatic world of qubits and quantum computing, one thing becomes clear: we stand at the precipice of a technological revolution. The dance of qubits, entangled and superposed, promises solutions to problems that once seemed insurmountable. Quantum computing’s potential to transform cryptography, optimize complex systems, and unravel the mysteries of our universe is both exhilarating and humbling.
In closing, we trust that this article has not only addressed your queries regarding “how is a qubit in quantum computing different from a regular bit in classical computing?” but has also sparked a deeper curiosity, encouraging you to delve further into this fascinating subject.
Iva Ort is an ingenious wordsmith and captivating blogger whose tales leap off the screen and into your imagination. With a pen as her wand, she weaves enchanting stories and insightful articles, leaving readers spellbound and craving more.